Introduction
Impact melt is a common product of hypervelocity impact events, generated from heat energy leftover from the recovery of shocked target rocks. These deposits comprise of either impact melt rocks (crystalline rocks with textures similar to plutonic (e.g., gabbro and granite) and volcanic igneous rocks (e.g., basaltic lava)) and/or glass and represent the whole-rock melts formed during the rapid shock-induced melting of target rocks that were in close proximity to the impactor.
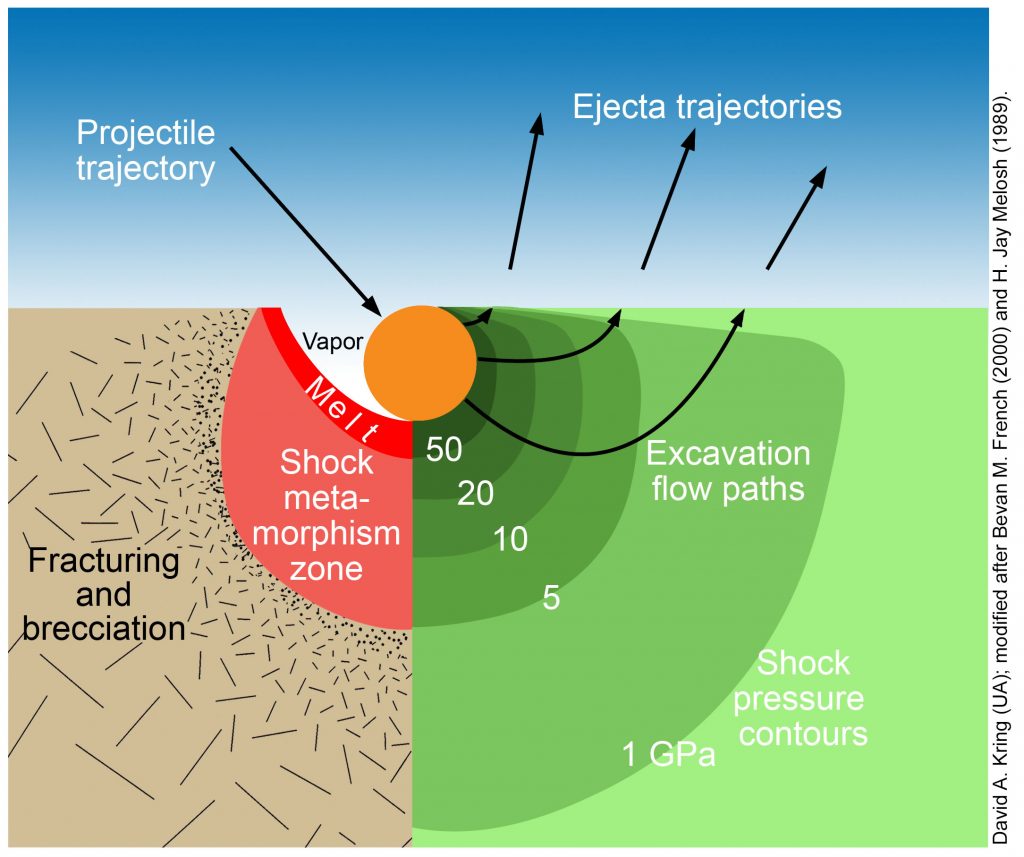
Early research on impact melt deposits stemmed from the first observations of the lunar surface from orbiter imagery. Smooth deposits were discovered within and outside of impact craters (inferred to be volcanic vents during the early to late 60s) on the Moon and they were interpreted to be melt produced from the intense and instantaneous release of energy from impact cratering events. During the Apollo missions, samples of lunar impact melt were returned to Earth for detailed analyses, and it was then scientists realized that impact melt deposits can be either chemically homogenous or heterogenous, and can experience multiple impact events, locking in the geologic history and age of various bombardment events. On Earth, impact melt was a popular research topic in the 1960s and 1970s at several Canadian impact structures such as Mistastin Lake, East and West Clearwater, and Manicouagan, where geologists studied the morphology, chemistry, and textures of impact melt deposits to establish a classification and nomenclature. However, our current understanding of impact melting is not yet complete, since most terrestrial impact melt outcrops have been heavily eroded by active dynamic processes such as fluvial, aeolian, glacial, volcanism, plate tectonics, vegetation, and sedimentation. On the Moon, impact melt deposits are relatively well-preserved (from what we can confirm from high resolution images acquired by cameras on spacecrafts such as the NASA Lunar Reconnaissance Orbiter), but we cannot obtain on the ground images, measurements, or samples at this time.
In Canada, there are teams of scientists and students working towards studying the impact melt process in the solar system and using terrestrial samples to better understand the formation and distribution of impact melt in and around lunar craters. Part of the Canadian Space Agency Lunar Exploration Accelerator Program, Dr. Gordon Osinski (Western University), Dr. Erin Walton (University of Alberta/
MacEwan University ), Dr. Chris Herd (University of Alberta), Dr. Roberta Flemming (Western University), and Dr. Myriam Lemelin (Université de Sherbrooke) are all leading teams to address high priority questions about lunar impact crater morphology, production of impact melt, and the effect shock metamorphism has on multiple types of lunar rocks.
Impact Melt Morphology and Distribution
Impact melt deposits can exist in a variety of structures and morphologies: (1) large kilometer-sized impact melt layers, (2) kilometer to meter-sized isolated bodies within the crater interior and rim region, (3) meter to centimeter-sized glassy lenses and particles mixed within impact breccias in and outside of craters, (4) meters- to centimeters-thick impact melt dykes intruding into the crater floor, central peak, and/or crater wall, and (5) discrete centimeter- to millimeter-sized glass particles distributed hundreds to thousands of kilometers from the crater center.
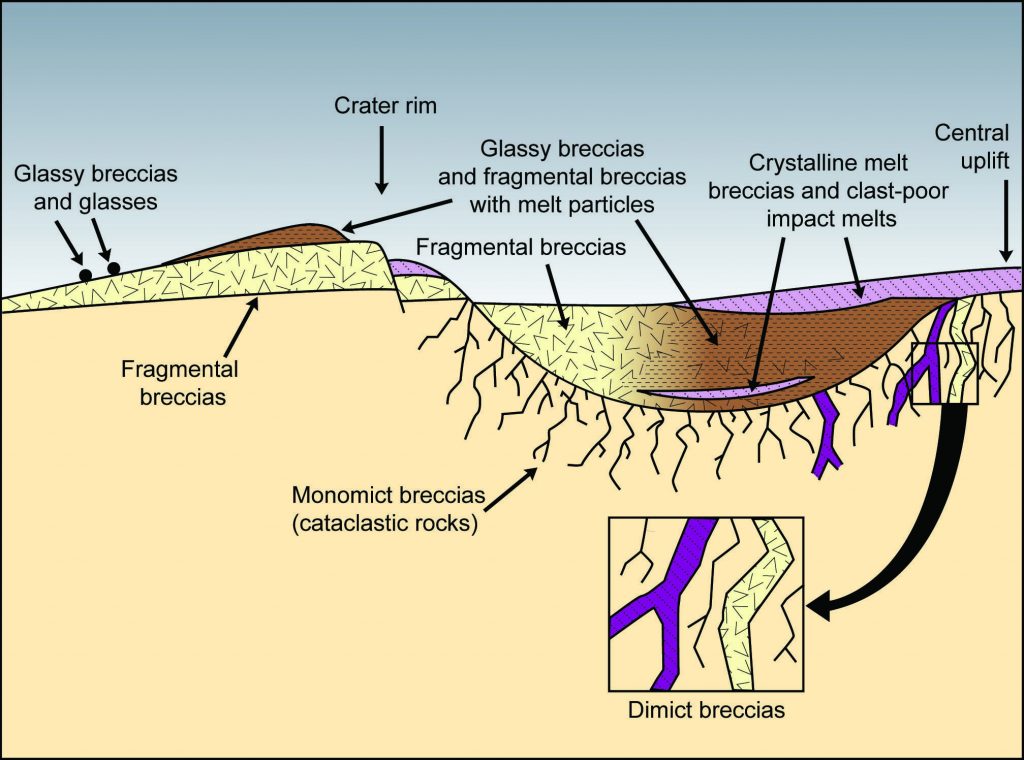
Crater Types
1. Simple Craters

In each type of crater, the structure and distribution of impact melt deposits is different, which is what prompts scientists at Western University and University of Alberta to study how these deposits form in all types of impact craters through field work and laboratory analysis. For simple craters, impact melt deposits primarily exist as isolated particles or lenses of impact glass mixed with impact breccias found within the crater interior (best example on Earth is Barringer/Meteor Crater in Arizona, USA). Isolated particles might be found on or ~0.5 crater radii away from the crater rim, but this is extremely rare. On the Moon, lava-like flows of impact melt have been found coming off the rims of simple craters, one example being the Korolev Z crater on the lunar farside (200.48°E, 1.15°N).
2. Complex Craters

In complex craters, impact melt deposits increase in size and volume compared to simple craters because the size of the impactor and the energy released during the impact event is greater. On the Moon, large ponds of impact melt are found covering the floor, terraces, and rims of complex craters, with some exhibiting impact melt flows. On Earth, impact melt deposits are found as impact melt layers and sheets, dyke systems, and isolated meter- to centimeter-sized particles in impact breccia deposits (known as impact melt-bearing breccias). Dyke systems and impact melt-bearing breccias are likely the most common impactite present on the Moon, but we cannot visually observe them using the high-resolution imagery currently available.
3. Peak-Ring Craters and Multi-Ring Impact Basins

Peak-ring craters and multi-ring impact basins contain the most impact melt by volume compared to any other type of impact crater. The amount of melt produced during these impact events is great enough to in-fill the crater interior and flow over the rim to fill topographic depressions surrounding the crater. The illustration on the left shows the extent to how much impact melt is produced from these impact events, and how deep target rocks are melted beneath the impact epicenter.
How Hot is Impact Melt?
One of the biggest questions in the impact crater research community is how hot does impact melt get when it forms and how long does it take to cool to a temperature where the melt and surrounding rocks reach an equilibrium. Since the 1960s, scientists proposed that due to the amount of energy released during impact crater events, impact melt forms under superheated conditions, where temperatures exceed the liquidus (temperature above when the entire material is liquid) of the target rocks. The temperatures proposed and accepted for over 5 decades ranged from 1700 °C to 2000 °C. In 2017, however, a study led by Dr. Nicolas E. Timms at Curtin University, Australia discovered that impact melt deposits from the Mistastin Lake impact structure exhibited superheated temperatures >2370°C, a few hundred greater than what has been accepted since the 1960s. Dr. Timms constrained this temperature from analyzing preserved crystal orientations within an accessory mineral known as zirconia (ZrO₂) found within a black impact glass sample. They used a technique known as electron backscatter diffraction to discover that this zirconia crystal once existed as its high temperature polymorph (mineral with a different structure but same composition), cubic zirconia (ZrO₂ in a cubic crystal structure).
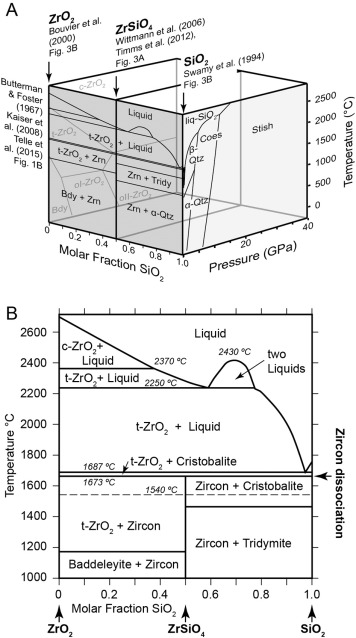
system. Phase diagram shows the polymorphs of zirconia and silica with the accessory mineral zircon (ZrSiO₄). Cubic-zirconia (c-ZrO₂) is found in low pressure and molar fraction SiO₂, and high temperature regions on the phase diagram. Credit: Timms et al., (2017).
Most recently, work by a team at the Institute for Earth and Space Exploration at Western University was able to confirm the temperature constraints reported by Timms et al., 2017 by adding four more data points, and also discovered that the preservation of superheated temperatures in impact melt deposits is most prevalent in environments where early quenching of melt occurred.
Dr. Gavin Tolometti, a planetary science postdoctoral associate at Western University published this new work (Tolometti et al., 2022), building on the long-term research goal to better constrain the temperature and pressure conditions of impact melt on all planetary bodies. Currently, Dr. Gordon Osinski at Western University, and Dr. Erin Walton and Dr. Chris Herd at the University of Alberta are working to apply the methods used by Dr. Timms and Dr. Tolometti to constrain the pressure and temperature of Apollo impact melt samples.
References
- Timms, N. E., Erickson, T. M., Zanetti, M. R., Pearce, M. A., Cayron, C., Cavosie, A. J., … & Carpenter, P. K. (2017). Cubic zirconia in> 2370° C impact melt records Earth’s hottest crust. Earth and Planetary Science Letters, 477, 52-58.
- Tolometti, G. D., Erickson, T. M., Osinski, G. R., Cayron, C., & Neish, C. D. (2022). Hot rocks: Constraining the thermal conditions of the Mistastin Lake impact melt deposits using zircon grain microstructures. Earth and Planetary Science Letters, 584, 117523.